Audio brought to you by Curio, a Lapham’s Quarterly partner
Not so long ago, at the start of 2007, the world’s population lived with a vivid technological divide. Half had a mobile phone: three billion people. Not quite a quarter used the internet. The phones were for talking. The internet required a computer. Wheelers and dealers—lawyers, agents, politicos—had BlackBerrys for emails, which they pecked on Lilliputian keyboards. But otherwise being online was a physically static condition. One surfed sitting still. The internet of the 2000s was an indoor child, happiest on the couch or behind a desk.
That changed the second week of that new year, when Apple CEO Steve Jobs teased the first iPhone from his jeans pocket, on a conference stage in San Francisco. Gaunt from the pancreatic cancer that would eventually kill him, he was nonetheless at the height of his powers as a technologist. The iPod, released in 2001, had been a phenomenon—a worthy follow-up to Jobs’ first great success, the original Macintosh computer, released in 1984. This new thing would be bigger than both, Jobs boasted. “Every once in a while, a revolutionary product comes along that changes everything,” he said from the stage. This was “three revolutionary products”: a phone, “a breakthrough internet communicator,” and a “widescreen iPod.” On a screen behind him, square pictographs of the trio spun into one another like a superhero changing costumes. “Are you getting it?” Jobs shouted while the audience tittered and then roared, wide-eyed at this shared moment of technological alchemy, of transmogrification, of near transubstantiation—all of which might sound purple except for everything that has come since.
It can be hard to recall now, but before Jobs’ black mirror, we were a species of button pressers. Nothing before came close to the iPhone’s fluidity and so seemed as much like the infinite tablet of a prophecy. Little surprise then that Apple has sold around two billion iPhones, making it among the richest companies in history, valued at more than $2 trillion. Forty percent of the world’s population now uses a smartphone. Democracy and truth have themselves been diverted by our phones’ pull on our attention, darkened in the shadow of our doom scrolling, unmoored in the weightlessness of our fiddling.
Occasionally, a singular technological leap changes what we expect from the world and the ability of human ingenuity to shape it. The iron horse of the railroad bent the geopolitics of the nineteenth century, just as the internal combustion engine, and the fossil fuel it requires, did the twentieth. It is a straight line from the Wright brothers’ 1903 biplane made of bicycle parts to the latest carbon-fiber Boeing. All defied what was then thought possible. Each seemed at first like magic, if of two different types. They start out nearly supernatural, a kind of witchcraft, but soon resemble stage magic, a refined trick built on years of practice and iteration. But whereas stage magic relies on sleight of hand, or some other subterfuge, the iterative magic of technology requires its inner workings to be revealed, exposed, and understood—in order to be refined, built upon, and made more marvelous and consequential. In An Enquiry Concerning Human Understanding (1748), David Hume notices that miracles no longer count as miracles when broadly seen. “There is not to be found, in all history,” he writes, “any miracle attested by a sufficient number of men, of such unquestioned good sense, education, and learning, as to secure us against all delusion in themselves.” Explanation ruins miracles. Familiarity deflates them.
Doing research on the web is like using a library assembled piecemeal by pack rats and vandalized nightly.
—Roger Ebert, 1998Technological magic is persistent. It stands up to scrutiny. It recharges overnight, cruises at highway speeds, and offers cocktails and in-flight movies. But Hume was right about the first impression of a miracle, that initial surprise and delight. There is a threshold between when a technology has to be imagined and when it is real enough to hold in your hand—a moment when the magical becomes real, when dreamed-of things finally happen, when a machine carries you into the sky, or a new medicine polishes a rough edge of human frailty.
Over this past year of human buckling, I have found myself craving that extreme ingenuity, those marvels that arrive infrequently but decisively. For a decade or two, it has become clear how desperately we need new energy technologies to provide the warmth, light, movement, and stuff we demand without catastrophically warming the planet. But this year brought a more specific and more desperate need: a microscopic technology to teach the body to fight the coronavirus, and the industrial and scientific infrastructure to manufacture it and deliver it into human arms. As vaccines have arrived, spectacularly if unevenly, one is tempted to be boastful: science did this. It is easy to worry that the miracle is, again, short-lived. But it has also made me wonder, What further leaps might we see? What else might be possible?
On the evening of March 18, 1987, the American Physical Society, physicists’ century-old professional organization, was midway through its annual meeting when the scientists in attendance crowded the hallways of the New York Hilton, eager to elbow their way into the big second-floor ballroom for a special evening session. The year before, two physicists, J. Georg Bednorz and Karl Alex Müller, had discovered that certain compounds of ceramic materials were remarkable “superconductors” of electricity: electrons flowed through them without any loss. Whereas resistance had been a given for any conductor of electricity—including the aluminum and copper used in power wires—these new ceramics had none, even at temperatures significantly higher than earlier superconductors. Condensed-matter scientists like Bednorz and Müller typically keep a lower profile than the astrophysicists or nuclear physicists accustomed to holding the spotlight with grand pronouncements about the nature of the universe. But in this instance, with these new superconductors raising the prospect of fantastic new applications—levitating trains, electric cars, new imaging tools like MRIs—the field went into a frenzy. Physicists around the world hoping to replicate (if not exceed) Bednorz and Müller’s superconducting success began testing new combinations of materials, looking for an organic mix that could begin superconducting at higher temperatures. Using the Kelvin scale (which starts at the scientific constant of absolute zero, or −273 degrees Celsius), Bednorz and Müller saw superconductivity at the then shocking 35 K (or −238 degrees Celsius). Soon others were leaping ahead, finding new material that worked at 38 K, then 52 K. According to Douglas Scalapino, now a professor emeritus at the University of California, Santa Barbara, it was as if everyone were running a four-minute mile: “You could go to any track meet, and some guy was breaking it.”
In that pre-internet era, with the rate of discovery outpacing the scientific publishing process, physicists were ravenous for news of the latest breakthroughs. Three thousand of them crammed into the Hilton ballroom for the High Temperature Superconductivity Symposium, while hundreds more watched on TVs set up in the hotel corridors. In a marathon session that soon became known as the “Woodstock of physics,” fifty-one separate presentations went on until 3:15 am, with example after example of new superconducting feats. It became a singular event in modern science, its legend fueled by a Nobel Prize that year for Bednorz and Müller and a cover story in Time magazine (“Superconductors!”).
Much of the hype was premature. Since these new superconductors were ceramics, rather than metals, they weren’t bendable like traditional conducting wires but instead were as brittle as dinner plates. To be useful, scientists—or really, engineers—had to manufacture these superconducting materials so that they could be coiled and wrapped. The practical applications would have to wait far longer than expected—not years but decades.
Not until Bob Mumgaard finished his PhD in applied plasma physics at the Massachusetts Institute of Technology, in 2015, did one particularly promising class of high-temperature superconductors made with material known as ReBCO—short for rare-earth barium copper oxide—reach a point of new potential. “The thing that really mattered was you could see this material in an adjacent field get better and better,” Mumgaard says, leaning toward me into his webcam one morning in 2020. Superconductors made from ReBCO operated at higher temperatures (100 K) and could be readily deposited into thin films that could in turn be wound into astonishingly strong and efficient electromagnets. Superconducting magnets had been used in hospital MRI machines and in the grand scientific experiments of particle accelerators, including the Large Hadron Collider outside of Geneva. But Mumgaard, a nuclear physicist, had locked in on their potential to fulfill the grandest promise of his field: fusion.
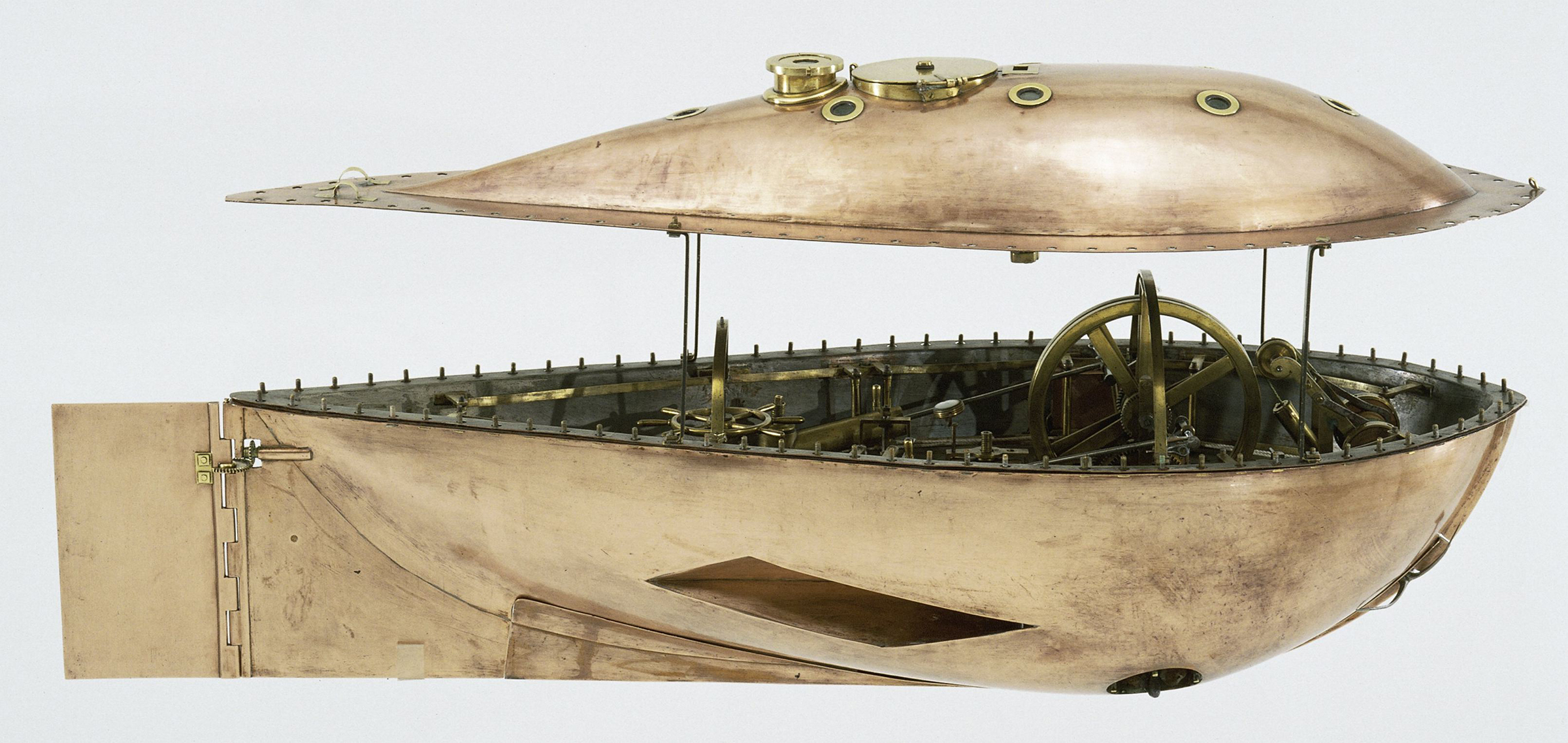
Copper model of a submarine, by Antoine Lipkens and Olke Uhlenbeck, 1836–39. Rijksmuseum.
The first extrasomatic energy source was fire, mastered by prehistoric societies 250,000 years ago. Pack animals provided ancient humans with an order of magnitude more energy. But not until waterwheels came into common use in the medieval era was there any common inanimate source to master. The Canadian historian Vaclav Smil notes that the Domesday Book records 5,624 water mills in southern and eastern England in the late eleventh century, one for every 350 people. Yet it would take another eight hundred years, into the Industrial Revolution, for their performance to be increased by another order of magnitude. Then things sped up. By the 1880s, the electrical system as we know it was recognizable, and crude oil began its rise to dominance for transportation. Ox by ox, waterwheel by waterwheel, engine by engine, the peak capacity of individual generating units rose approximately fifteen million times in ten thousand years, with more than 99 percent of that rise occurring during the twentieth century. Of those leaps, the most dramatic was nuclear fission, the breaking apart of atoms. Fission weapons shaped the century’s geopolitics; fission power plants still supply 10 percent of the world’s electricity.
Except now fission has run its course. In the wake of the Fukushima disaster, society’s appetite for nuclear risk has diminished. The costs of engineering even greater safety make fission power less economically viable compared to the falling costs of renewable sources like wind and solar. Averting further climate catastrophe requires broad policy changes—and some key new technologies. A step-change improvement in energy storage would open up new ways of using renewable energy, like solar energy at night and wind energy on calm days. More efficient ways of removing carbon from the atmosphere, at scale, might help change the climate again.
But the greatest potential for innovation—the closest thing to a technological silver bullet—remains fusion. Fusion is what powers the sun: a self-sustaining reaction in which isotopes of hydrogen at tens of millions of degrees fuse to form helium, releasing vast amounts of energy in the process. Fusion carries none of fission’s catastrophic risks. Its raw materials are abundant and safe, derived primarily from seawater. Its waste is minimally radioactive—more like what’s produced by hospitals than fission power plants. And there is no risk of meltdowns: when a fusion reactor’s power is shut off, its reaction stops.
The challenge is a different kind of control. Fusion reactions take the form of a roiling hot plasma, burning at more than 50 million degrees Celsius. Engineering its containment—putting “the sun in a bottle,” in a classic metaphor—has consumed scientists since the 1950s. The leading strategy is a type of reactor known as a tokamak, a doughnut-shaped chamber that uses electromagnets to hold the plasma in place. Since the tokamak was conceived in 1950, by the Soviet scientist Andrey Sakharov, the stumbling block has been finding magnets powerful enough to hold the plasma but efficient enough to require less energy than the fusion reaction itself creates. (Otherwise what, ultimately, is the point?) That’s where superconductors come in. “If you can figure out how to build a magnet out of this material, the material itself stops being the limit, and instead the engineering becomes the limit,” says Mumgaard. “And if you can do that, you can make small fusion reactors, and you can do that without having to have some big scientific breakthrough in plasma physics.” A working fusion reactor has been perennially out of reach. But a proper magnet made of superconductors offers a new path.
Inventor, n. A person who makes an ingenious arrangement of wheels, levers, and springs and believes it civilization.
—Ambrose Bierce, 1911Mumgaard cofounded Commonwealth Fusion Systems in 2018, almost as soon as he deemed the technology ready for his uses. Throughout graduate school, he had kept a close eye on the progress of thin-film manufacturing, the process needed to shape superconducting ceramics into useful forms. For other thin-film products like solar panels and silicon computer chips, enormous economies of scale led to constant technological improvements. If the same could be done for ReBCO, then it could be worked and coiled into extremely powerful electromagnets—powerful enough, perhaps, to be fusion’s missing piece.
In 2021 Commonwealth will begin construction on a new headquarters campus, designed to accommodate the fabrication and testing of a two-step project. The first is a massively powerful magnet made with high-temperature superconductors. The second uses the magnet as the transformative component in a fusion reactor capable of producing more energy than it requires to operate. Called SPARC, it is especially remarkable for its relatively small size, with the entire unit taking up about the same space as a volleyball court. This is startlingly intimate in contrast with the grand scale of other fusion projects, most notably ITER, an international collaboration currently under construction in the South of France. Designed over decades, beginning in 1988, ITER’s tokamak doesn’t reap all the benefits of high-temperature superconductors, requiring its magnet to compensate with sheer size in order to generate enough force to contain the plasma. Its budget has stretched into the tens of billions of dollars (the precise number is a matter of considerable dispute). Despite beginning construction in 2013, its first fusion reaction is not expected until 2035.
For Mumgaard (and perhaps all of us), that is too late to be useful. On their current timeline, he and his colleagues hope to press a button that kick-starts a fusion reaction sometime before 2025. When they press it again, the reaction will stop. The net energy created will be the first glimmering light of—quite literally—a human-made sun. “We think it will be a really, really big deal,” he says with quiet understatement. “It’s already a big deal—fusion made all the atoms and all of us, right? It’s the most powerful thing in the universe: 99.999—all the way out—percent of all the energy in the universe starts with fusion.” Once that sun can be turned on and off at will, the challenge will be to extract its excess energy and package it into something resembling a power plant. Then build them, fast.
It is easy to be defeatist about climate change. The political dread of the past several years, the wildfires, the thawing permafrost, the unrelenting virus, make the specter of extreme disruption familiar. But to take just one slice of humanity’s challenge—halting the burning of fossil fuels—it seems possible, with a squint, to see this as a solution. Mumgaard likes to imagine the fuel truck backing up to the plant on the first day and pumping the totality of the hydrogen isotopes, delivered as a gas, required for its entire working life. No coal trains, no tank farms, no underground pipelines of rushing hydrocarbons. In the years following, one imagines, thousands of fossil-fuel power plants that dot the planet, emitting the gases that are cooking us all, can be replaced by thousands of fusion reactors. These would not displace the enormous progress already made with renewables but compensate for their limitations. To create a reliable electric grid exclusively out of variable generation—that is, dependent on the wind or the sun—means building the excess capacity required to cover calm or cloudy days, along with enormous batteries to cover the gaps and the nights. It is prohibitively inefficient. We need “dispatchable” power—easy on, easy off. Fusion could be that, and then some, eliminating (or minimizing) the need for nuclear fission, as well as fossil fuels.
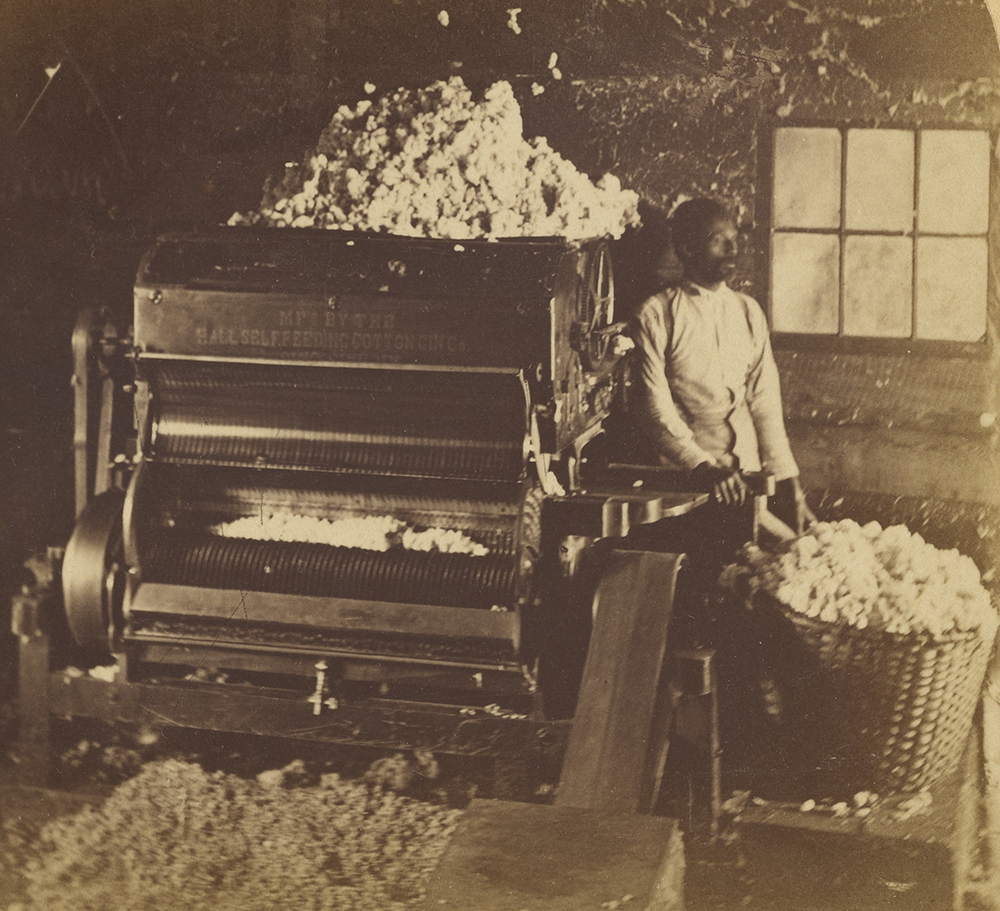
A Cotton Gin, by Franklin G. Weller, c. 1870. The J. Paul Getty Museum, Los Angeles. Digital image courtesy the Getty’s Open Content Program.
If fusion works, the world will change. The hydrocarbons that established society as we know it will be replaced with a clean power source—a magic, at least for a moment, as if out of science fiction. (In the original Star Trek, first aired in 1966, the power source was described as a kind of fusion.) What then unravels? And what newly forms? Freshwater would be more abundant if the energy to desalinate it were, too. Absent the cost of power, more products and materials could be recycled economically, opening up new possibilities for circular material flows. It’s a tantalizing vision, and also totalizing. Fossil fuels have long been the scaffold of the global economy, but what happens when they are plausibly removed from the equation? “The two fundamental markets are human creativity and energy,” Mumgaard says—it’s the second of ten mantras by which Commonwealth guides itself. (Number one: “Energy and a livable environment are both fundamental human rights.”)
But what’s startling to me is that, as implausible as it sounds, transcendent, magical inventions have happened before. Fusion has always emitted the peculiar energy of magical thinking, even stranger as it gets closer to reality. What happens when the magical becomes real? When people fly through the air, when books light up with infinite knowledge, when energy is limitless? At the least there is hope in this space of possibility—this wide gap where reality and fantasy join. It is an abiding thrill of technology that there are moments when it asks us to look away from its externalities—from the conflict minerals, the emissions, the noise, the ad-supported models that suck our attention, destroy the livelihood of culture producers, and warp politics—and toward a miraculous, or just plain livable, future.
We are living on the knife edge of all that now. Will the vaccines beat the virus? Will the technological alternatives to fossil fuels come fast enough to limit the suffering—and perhaps the ultimate apocalypse—of climate change? The promise of technology is the possibility of all its little innovations, those miracles that become commonplace, to amount to the grand magic of ongoing life on earth.
from Hacker News https://ift.tt/3rBnrAx
No comments:
Post a Comment
Note: Only a member of this blog may post a comment.